27. Color
Summary
Color
The visible light section of the electromagnetic spectrum encompasses light waves from red to violet. Perhaps you’ve heard the acronym ROYGBIV which stands for red, orange, yellow, green, blue, indigo, and violet. While visible light is better described as a spectrum rather than as discrete quantities, we consider ROYGBIV to be the major colors of visible light. What distinguishes each color is the wavelength of that light. Red light has long wavelengths, green is in the middle, and violet has the shortest wavelengths of visible light. The table below gives the approximate wavelength ranges for each color of visible light.
color |
wavelength range |
red |
700 – 640 nm |
orange |
640 – 590 nm |
yellow |
590 – 560 nm |
green |
560 – 500 nm |
blue |
500 – 450 nm |
violet |
450 – 400 nm |
Human eyes contain two types of light receptor cells. They are called rods and cones (based on the shapes of these cells). The rods are sensitive to dim light but not to color. The cones are what allow us to perceive colors. There are three types of cone cells: one sensitive to short wavelengths of light, one sensitive to medium wavelengths, and one sensitive to long wavelengths. However, the cones require higher intensity light in order to “fire” and send a signal to the brain. This is why we can see objects in a very dimly lit room, but we cannot distinguish colors very well. Also, when we look at the night sky with the naked eye, almost all the stars appear white. The light intensity of the starlight can trigger the rods, but does not have enough intensity to trigger the cones. When we look at stars through a telescope, the increased light gathering of the instrument will then allow us to see the vast variety of star colors. When light waves of different wavelengths and intensities interact with our rods and cones, we perceive things as taking on different colors as a result. Physical or inherited issues with cone cells can be a cause of color-blindness.
The three primary colors of light are red, green, and blue. Using color addition, which will be discussed later in this chapter, these three colors alone can be combined in varying intensities to generate all other colors of visible light.
Transmission, absorption, and reflection
When we look at objects, we don’t actually see the objects themselves. We see the light that reflects off of them into our eyes. In the case of transparent objects, we see the light waves that transmit through the objects.
Reflection is what occurs when waves bounce off of a surface. When we look at something blue, that means that blue light is reflecting off the object into our eyes. Red and green light waves are absorbed by the object, which is why we don’t see those colors. A red object reflects red light and absorbs blue and green light.
Absorption occurs when a material absorbs the energy from a light wave, preventing it from either reflecting or passing through that material.
In general, objects that reflect light into our eyes reflect the colors that we see and absorb the colors that we do not see.
Transmission occurs when light waves pass through an object. When we look through blue transparent objects, those objects transmit blue light and absorb green and red light. When we look through a yellow transparent object, it transmits red and green light and absorbs blue light.
Other objects that we see via transmission of light are light sources themselves. This includes LEDs, street lights, and some light bulbs. Red light sources transmit red light waves to our eyes, green light sources transmit green light waves to our eyes, and so on.
Objects that we see via light transmission transmit the colors we see and absorb the colors that we do not see.
Color addition
Color addition refers to the ability to use light to create secondary colors from primary colors. Mixing various intensities of red, green, and blue light allows us to create any other color of light.
Color addition is related to light we see via transmission. When two primary colors are transmitted together, we see a secondary color. When red and blue of equal intensity are added, a pinkish-purple called magenta is created. When red and green of equal intensity are added, yellow is created. When blue and green of equal intensity are added, cyan is created. Magenta, yellow, and cyan are therefore known as secondary colors. When all three primary colors are added at equal intensities, the result is white light. This is sometimes referred to as the RGB (red-green-blue) color model, and is shown in Figure 27.1. All other colors can be generated with mixtures of red, green, and blue light at different intensity levels.

Images, websites, and videos that are viewed on computers, TVs, and other electronic devices are defined with an additive color model. An LED display contains tiny pixels of red, green, and blue LEDs (an example of which is shown in Figure 27.2).
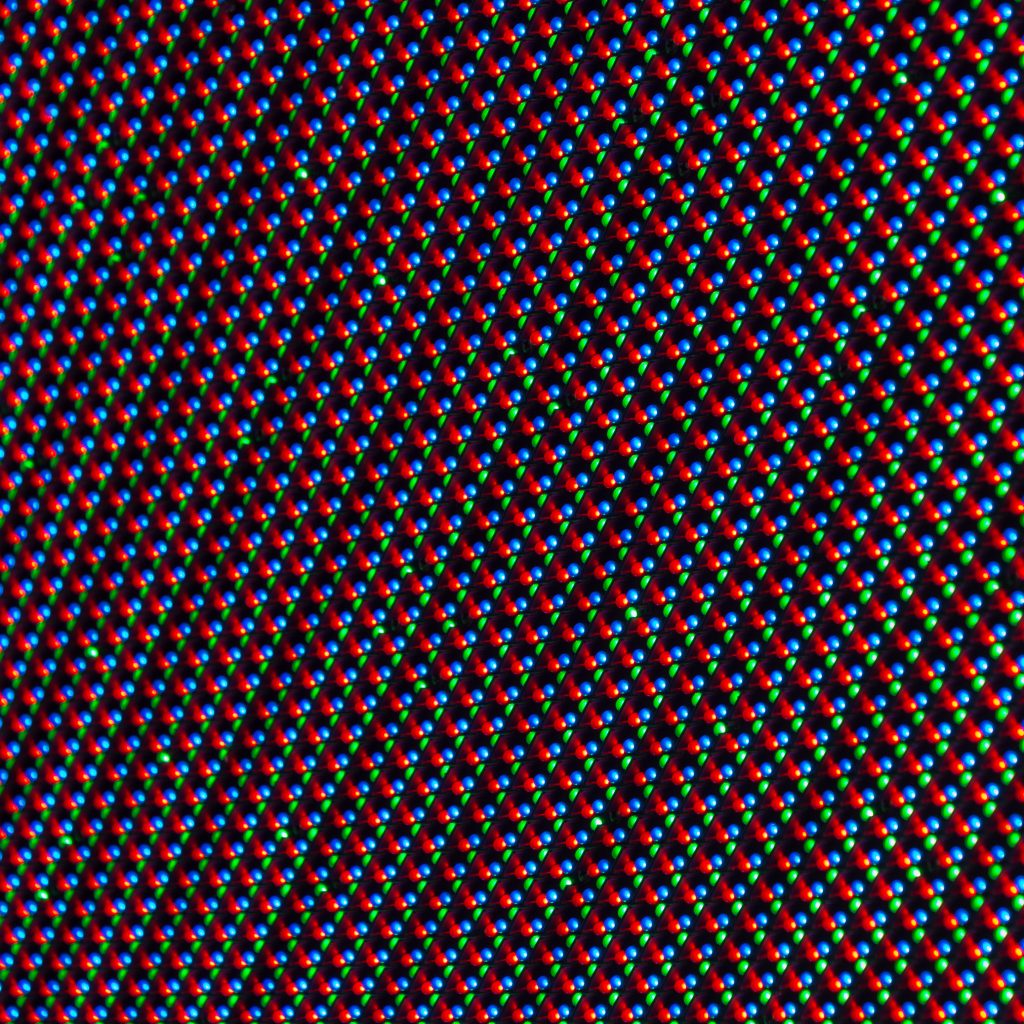
Many LCDs (liquid crystal displays) contain a white backlight that provides background illumination. This light is then sent through red, green, and blue filters to generate the colors you see on a television, smartphone or tablet screen, or computer monitor.
In the video below, Dr. Pasquale uses RGB light-emitting diodes to demonstrate color addition. The top dial changes the intensity of the red light; the center dial changes the intensity of the green light; and the bottom dial changes the intensity of the blue light. When Dr. Pasquale turns on the red and green LEDs, the result is yellow. Red and blue generates magenta light. Green and blue creates cyan light. Finally, Dr. Pasquale turns on all three colors and the result is white light.
When it comes to light and color addition, white, as mentioned, is generated when all primary colors are mixed at equal intensities. The color black is perceived when there is no light at all. In that respect, black can be considered the absence of colors.
Color subtraction
While color addition pertains to light transmission, color subtraction pertains to light reflection. Subtractive color models are used primarily in print. When you look at a color magazine, book, or poster, you are seeing the light waves that reflect off of the pages and into your eyes.
Color subtraction refers to the colors that are absorbed by pigments or other printed material. Cyan absorbs, or subtracts, the color red. Magenta absorbs the color green. Yellow absorbs the color blue. Secondary colors therefore subtract out primary colors. Through this color subtraction, other colors can be generated.
When cyan and yellow are mixed in a subtractive model, blue and red are subtracted and the result is green. When cyan and magenta are mixed, red and green are subtracted and the result is blue. When magenta and yellow are mixed, blue and green are subtracted and the result is red. When cyan, magenta, and yellow are mixed, the result is black. This is sometimes referred to as the CMY (cyan-magenta-yellow) model, and is shown in Figure 27.3.

Color printers work by using separate cyan, magenta, and yellow inks. Usually, a separate black ink is used to generate black without using excessive amounts of cyan, magenta, and yellow ink. If you’ve ever heard or seen the term CMYK, that refers to subtractive colors used in printing: cyan, magenta, yellow, black.
While color subtraction is a model that explains color printing, it is not well-suited to explain color mixing of paints. The color of two mixed paints depends on the chemistry of the pigments themselves as well as the thickness of the paint, and requires very complicated mathematics to approximate (source [PDF, 2 kB]).
Scattering
Selective transmission, reflection, and absorption explain the colors of most things we see on this planet. But some phenomena require the concept of scattering to explain. Scattering is a process in which the direction that light travels is changed without affecting the wavelength of the light.
For example: why is the sky blue on Earth? The Earth’s atmosphere is composed of many particles of different gases, as we discussed earlier in this textbook. When light from the Sun travels through these particles, due to the size of the particles, short wavelength light is scattered more efficiently than longer wavelength light. Atmospheric scattering causes short wavelength light such as blue and purple to reach the surface of the planet. Our eyes are more sensitive to blue than purple, therefore we perceive the sky to be blue. The blue sky as seen from the Adirondack Mountains of New York State is shown in Figure 27.4.
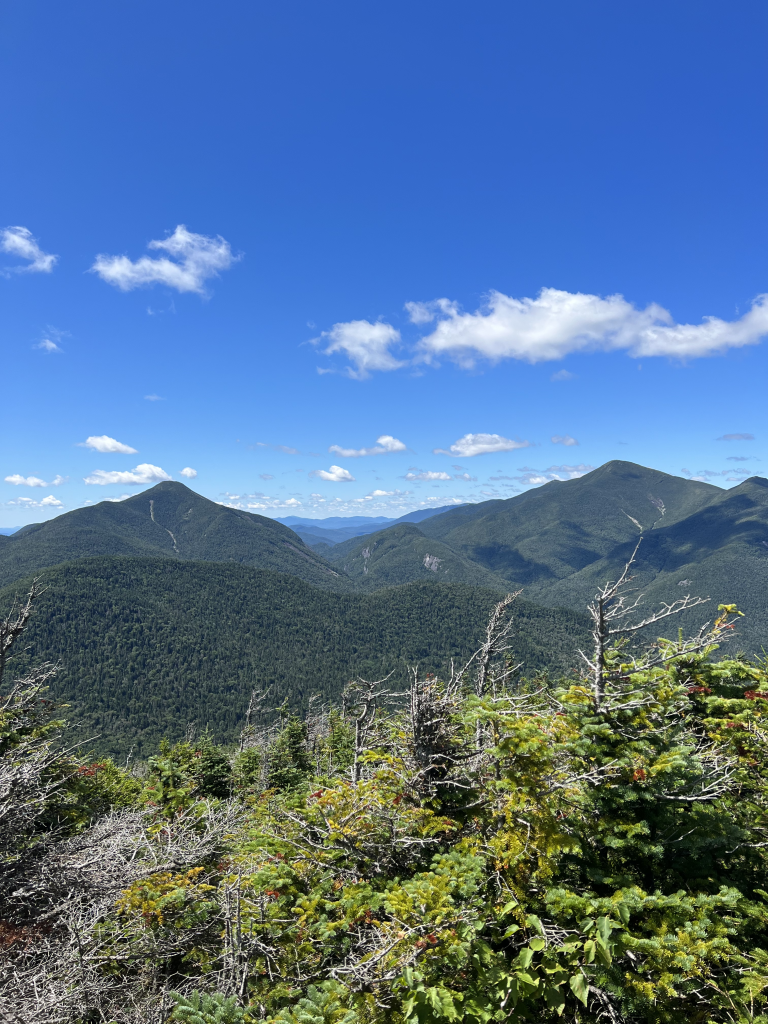
Cloud particles, being larger than atmospheric particles, scatter longer wavelengths as efficiently as shorter wavelengths. This means that essentially all wavelengths of visible light are scattered equally well. This makes fair-weather clouds appear to be white, as seen in Figure 27.5.
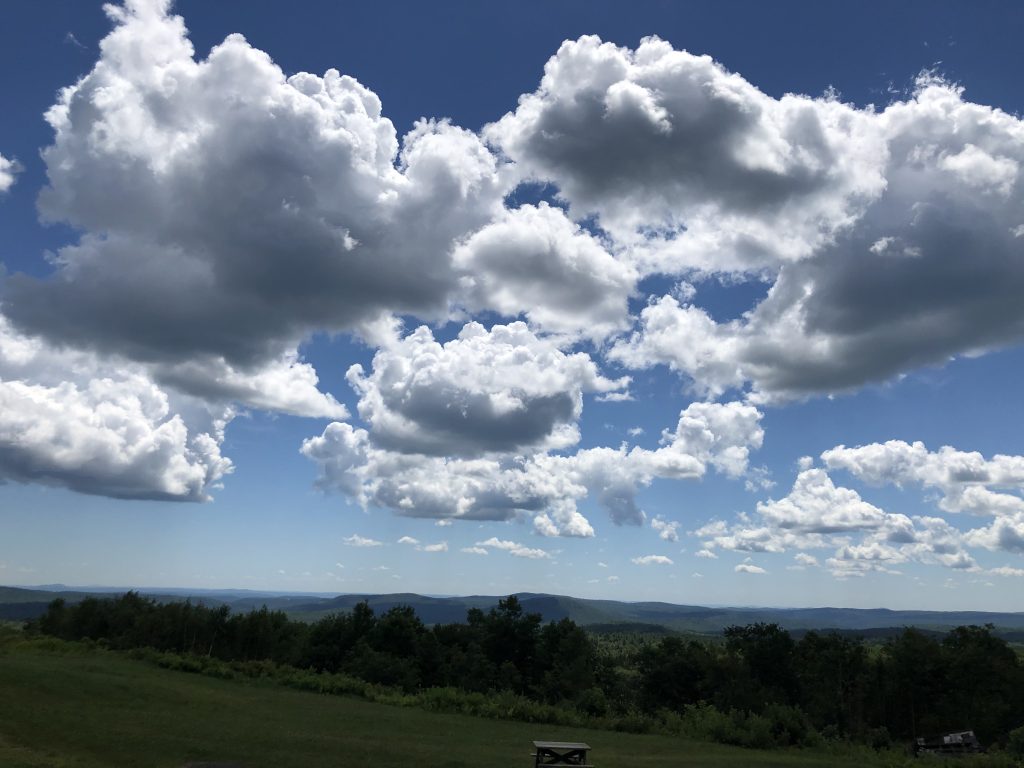
On overcast days, the sky appears gray, a muted shade of white. A gray overcast sky over Herrick Lake Forest Preserve is shown in Figure 27.6.
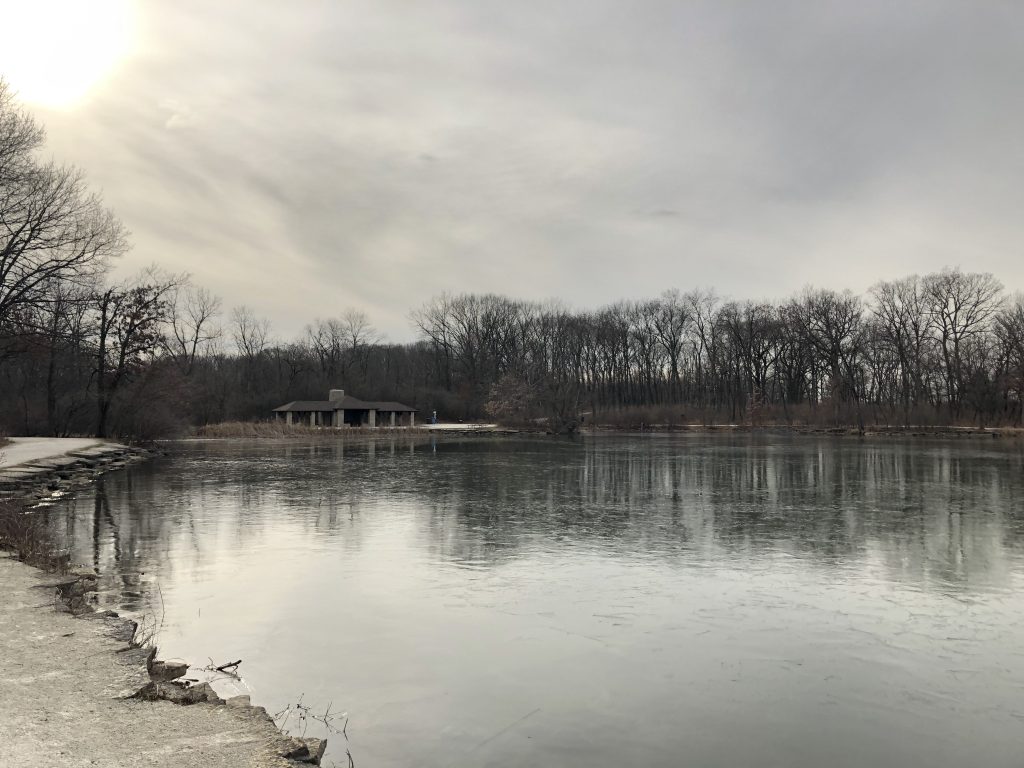
During sunrise and sunset, the Sun’s shallow angle with respect to the horizon means that light from the Sun has to travel through more atmosphere than it does at other times of day when the Sun is high in the sky. When sunlight travels through so much atmosphere, so much blue light is scattered away that only the longer wavelengths of visible light are able to reach our eyes. The effect is a red sky. Sunset’s striking colors, as seen from the College of DuPage parking lot, are shown in Figure 27.7.
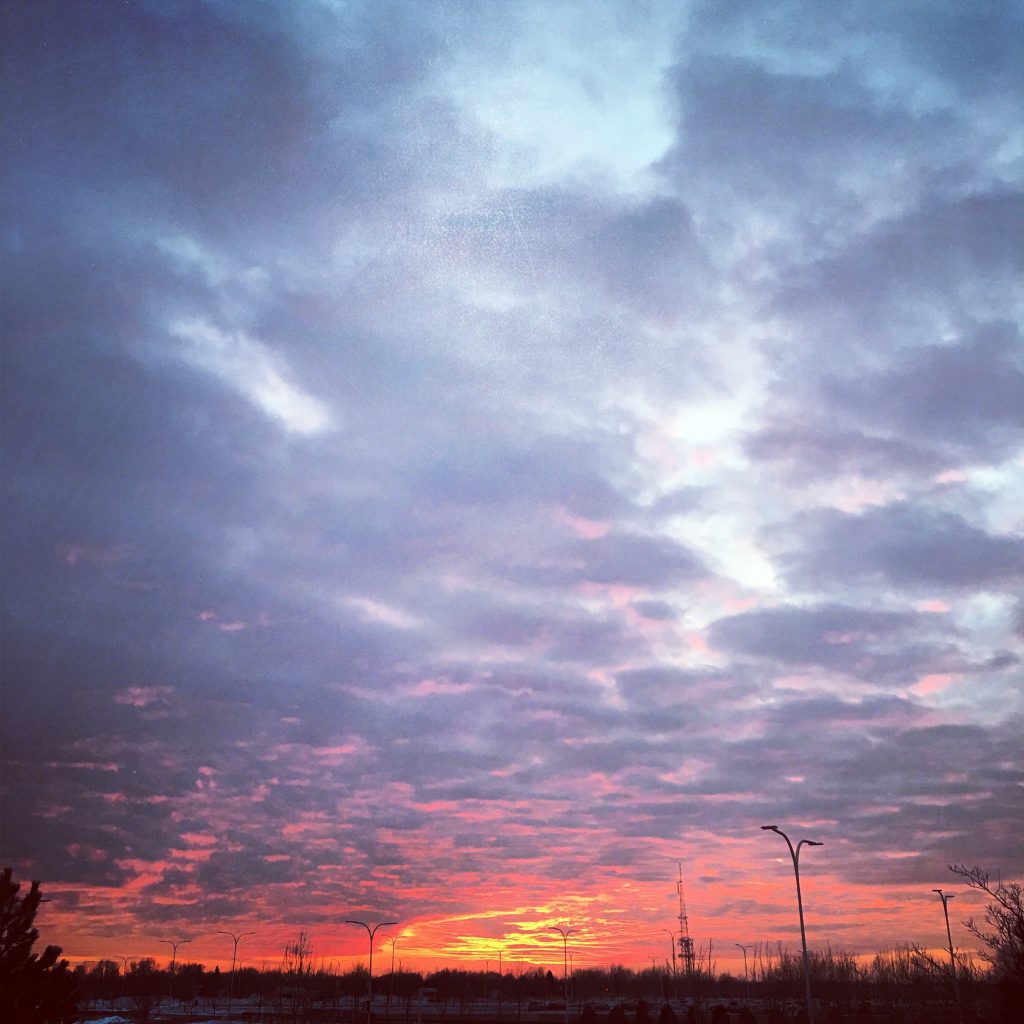
In the video below, a fish tank is filled with water. Dr. Fazzini shines a flashlight inside to demonstrate how light passing through the clear water looks from both the side and head-on. The clear water does not noticeably scatter any of the light as evidenced by the fact that the beam is not visible when viewed from the side. As a result, the light that passes through appears white. After filming that part of the video, milk was mixed with the water to act like the molecules in our atmosphere. Dr. Fazzini again shines the flashlight into the fish tank. The flashlight acts like the Sun, providing a white light source. When the flashlight is directed into the milk-water mixture, the visibility of the beam when viewed from the side demonstrates scattering of the light. The short wavelengths of light (notably blue) are scattered the most. When viewing the direct beam, the long wavelengths of light (orange and red) are what remain after the short wavelengths have scattered out. This is similar to what happens at sunrise and sunset.
If the color of the sky is explained by our atmosphere, then what happens on celestial bodies with no atmosphere? On the Moon, for example, there is no atmosphere to scatter any wavelengths of light. The Sun appears as an orb in space, and the surrounding areas of space are black, as seen in Figure 27.8. Photos from Moon landings depict the darkness of space. Note that in Figure 27.8, the presence of glare is likely due to the astronaut’s helmet and there additionally appears to be lens flare caused by the camera. None of those optical effects is due to atmospheric effects, as the Moon has no atmosphere!
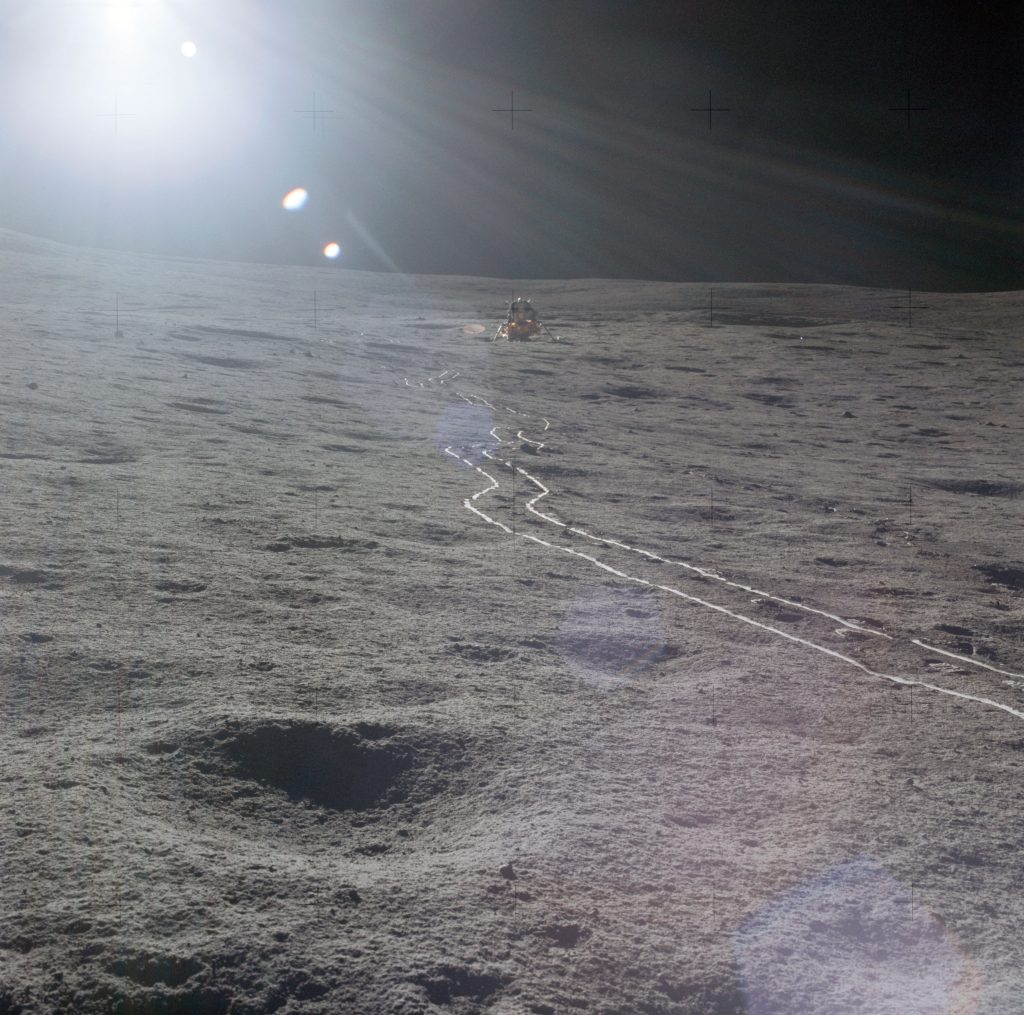
The atmospheric composition of Mars leads to a brownish pink sky color. Mars landers have taken color photos depicting this fascinating sky color. A color photograph of the Mars lander Ingenuity, surrounded by the Martian surface and sky, is shown in Figure 27.9.
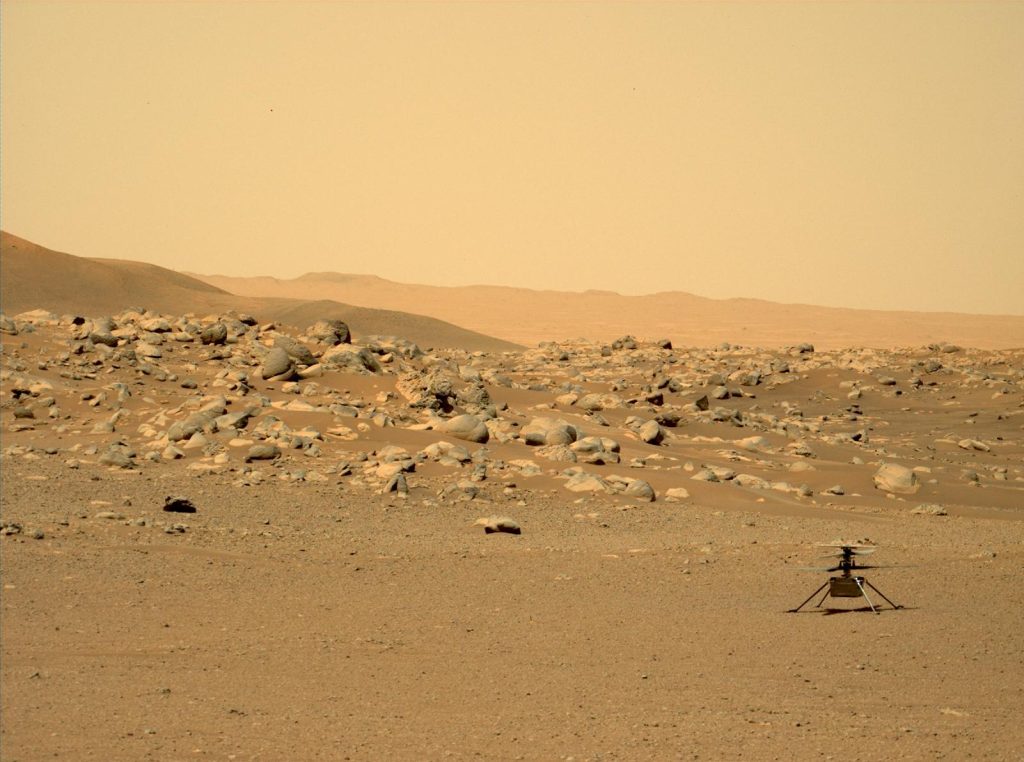
Further reading
- Color wheel – Learn about color theory and what colors look good together and why.
- Coblis Color Blindness Simulator – This simulator can show you how different images appear to individuals with different types of color vision deficiencies. (In particular, it is a helpful tool for ensuring that images and graphics are accessible.)
- Luftwerk Color Code – This art installation, which was exhibited at the College of DuPage’s Cleve Carney Museum of Art in 2017, uses the effect of different color lighting on different color paints to achieve different artistic results. A video interview with the artists demonstrates many of these visual effects.
Practice questions
Conceptual comprehension
- If you shine equal intensities of red light and green light onto the same spot, what color will you perceive? Why?
- Using color addition, how would it be possible to generate colors such as orange or purple, which are not secondary colors of light?
- Imagine you have a red filter and a blue filter. If you look through both filters simultaneously, what color will you see? How does this relate to the concept of color addition?
- If you shine white light through a green filter, what color of light will appear to transmit through? Why? How would this change if the light is red, green, blue, or a mixture of those colors?
- If you shine white light onto a surface covered with a cyan object, what color will the object appear, and why? How would this change if the light is red, green, blue, or a mixture of those colors?
- Consider a scenario where you have a blue object under red light. What color will the object appear? Why?
Hands-on experiments
- Use flashlights with colored filters or cellophane (red, green, blue). Shine each color onto a white surface and observe how they combine when overlapping. Then, shine each color onto different colored surfaces or objects and observe the results.
- Place objects of different colors (such as toys, fabrics, or even colored candies) in direct sunlight. Observe how the colors appear. Then, bring the objects indoors and compare the colors that you see. Is indoor lighting the same or different from sunlight?
The electromagnetic spectrum describes the entire range of electromagnetic waves. Each subset of waves can be defined in different categories based on its frequency or wavelength.
The wavelength of a wave describes the shortest distance between two identical repeating points on a wave. (symbol: λ, unit: m)
Intensity describes the amount of energy per unit of area that reaches a certain location every second (unit: W/m^2)
Color addition refers to the ability to use light to create secondary colors from primary colors (red, green, and blue).
An object is transparent if it allows visible light to pass through without getting scattered or absorbed.
Reflection occurs when a wave bounces off of a surface and subsequently changes its direction.
A wave is a periodic oscillation that transfers energy from one place to another.
Absorption of light occurs when light waves completely transfer their energy to a medium as they attempt to pass through it. The light is therefore blocked from transmitting through the medium.
Energy is defined as the capability of an object (or collection of objects) to do useful work. (symbol: E, unit: J)
Transmission occurs when light waves pass through an object.
A diode is a circuit element that acts like a one-way valve in that it only allows current to flow in one direction but not the other.
Color subtraction is a color model based on light reflection and absorption. Colors that are not reflected back into our eyes are subtracted out and modify the color that we perceive.
Scattering is a process where the direction that a wave travels in is changed without affecting the wavelength.
Gas is a state of matter where molecules are very free to move about and generally do not interact with each other except during collisions. This means that the shape and volume of a gas is free to change. Gas is one of the four most common phases of matter.