17. Phase change
Summary
Phases of matter
In our everyday lives, we experience four states of matter: solid, liquid, gas, and plasma.
A solid is a material where the molecules or atoms are very tightly bound together. This gives a solid a very rigid volume and shape. A solid can therefore be defined by the fact that a solid will not change either its volume or its shape.
A liquid is a material where the molecules or atoms are free to move about much more easily than in a solid, however, the molecules are still packed rather closely together. A liquid will change its shape, but cannot be easily compressed to change its volume. When pouring liquid from one container into another, while the shape of that liquid will change, the volume of the liquid will not change.
In a gas, the molecules are very free to move about, and they are not arranged tightly at all. This means that the shape and volume of a gas is free to change. A gas will assume the same shape as the container that it’s in, and can be compressed to take up less space, or expanded to take up more space. If you’ve ever used an air compressor to fill up your car or bicycle tires, then you’ve changed the volume of air.
A plasma is an ionized gas. That is to say, it’s a gas with so much energy that electrons have been ripped away from the atoms. While this might seem like an exotic state of matter, it’s something we experience in our daily lives. Stars, including our Sun, are made of ionized hydrogen and helium: plasma. Lightning is a plasma. Even fluorescent bulbs use a plasma to create light.
In general, we can characterize each state of matter by the amount of energy it has. For any given substance, a solid has the least amount of energy, followed by a liquid, followed by a gas, and then plasma, which has the most energy.
While there are four common states of matter, there are in fact a lot of other states of matter. However, they tend to be very exotic. That means they need to be under very special situations to occur. Some states of matter require temperatures very close to absolute zero, or require extraordinarily high pressure to exist. We are very unlikely to ever deal with these states of matter in our day-to-day lives.
Exotic states of matter
States of matter that exist at extremes of temperature and pressure have been discovered throughout the years.
Superfluids, which are created when certain liquids are cooled to extremely low temperatures, experience zero viscosity. This enables them to flow through pores in otherwise solid objects (such as ceramic) and climb the walls of containers and flow to the lowest point.
Bose-Einstein condensate is a state of matter that is also achieved at extremely low temperatures. In this type of matter, quantum physics properties that are usually only observed on extremely small scales become apparent on much larger scales.
There are many other types of states of matter, both experimentally validated and theoretically proposed by scientists. These exotic states, such as those found in neutron stars or in the earliest seconds of the universe, provide fascinating research opportunities for physicists interested in matter.
Vaporization and condensation
Vaporization is the phase change that occurs when a substance converts from a liquid to a gas. Because a gas has more energy than a liquid, that means that energy must be provided to a substance to get it to change from a liquid to a gas.
In the vaporization process, molecules in their liquid state absorb energy. That energy loosens the bonds between the molecules, freeing them to move about as a gas. Heat is required to convert a liquid to a gas. That heat must come from somewhere. When vaporization occurs, the surrounding environment cools down after it provides that heat. It is important to note that because the surrounding environment is typically extremely large with respect to the substance that is vaporizing, the temperature change of the environment is typically not noticeable. (This is similar to the example earlier in this textbook when we discussed how dropping a smartphone will cause the Earth to accelerate upward, but to such a small degree that it can be essentially ignored.)
There are two types of vaporization: boiling and evaporation. Boiling will be discussed in the next section of this chapter. Evaporation is a subset of vaporization that occurs on the surface of a liquid. That is, even if we have a large volume of liquid, evaporation only occurs at the interface between the liquid and surrounding fluid, such as air. To demonstrate this, in the video below, Dr. Pasquale sprays a small amount of alcohol onto a lab bench and places a fan nearby. The alcohol evaporates by changing phase to a gas at the surface. The pool of liquid becomes shallower and shallower until all of the alcohol is converted into a gas.
Evaporative cooling is something that we as humans experience frequently. Our bodies sweat when it’s warm. Our skin provides the heat required for that sweat to evaporate, causing our skin to cool down. Evaporative cooling can also be used in homes in areas with a dry climate. In the Chicagoland area, evaporative cooling is not as effective as air conditioning due to our humid summer climate.
To demonstrate that evaporation is a cooling process, in the video below, Dr. Pasquale dips a thermometer in a bottle of alcohol and then waves it around in the air to get the alcohol to evaporate. Heat from the thermometer changes the phase of the alcohol from a liquid to a gas, and as a result the temperature recorded by the thermometer decreases.
The drinking bird (shown in the video below) is another example of the cooling properties of evaporation. The bird’s head is dipped into water. As the water evaporates from the bird’s head, the cooler temperature causes the pressure inside the head to decrease. This pressure differential causes the fluid inside the bird’s body to be drawn up into the head, causing a net torque. A net torque will create rotational acceleration, causing the bird’s head to rotate downward. The bird dips its beak and the fluid in the head is able to travel back into the bottom of the bird. This process repeats until the bird’s head dries off and evaporation stops.
Condensation is the opposite of vaporization. It is the phase change from a gas to a liquid. As the molecules lose energy in the condensation process, they start to assemble in a tighter form, which gives liquids a more well-defined arrangement than gases. The energy lost by the substance is released into the surroundings, causing them to warm up. (Again, as the surrounding environment is typically very large compared to the condensing substance, this does not usually cause a noticeable temperature change.)
Condensation is something you may have noticed if you have a cold glass of water, or can of soda, sitting out on a warm or hot day. The water vapor in the air surrounding the container will condense into a liquid form, causing beads of liquid on the container (Figure 17.1).
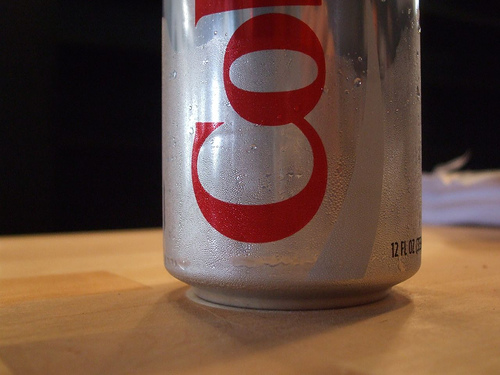
It is important to note that, for a given substance, vaporization and condensation occur at the same temperature. Pure water at 1 atm of pressure will vaporize at 100 oC, and it will also condense at 100 oC. Whether it converts from a liquid to a gas, or from a gas to a liquid, depends on whether it is gaining or losing energy at this temperature.
Boiling
Boiling is a type of vaporization (change of state from liquid to gas) that occurs throughout the volume of a liquid. Boiling occurs when the average kinetic energy of the molecules in the liquid becomes large enough for the molecules to start to overcome the cohesive force that keeps them attracted to each other. When this occurs, molecules in the liquid separate from each other and convert into the gaseous form as they remain situated throughout the liquid. Eventually, the buoyant force or kinetic energy of the gas bubbles will cause them to rise up and out of the liquid. That is, bubbles of gas are able to form without being squashed out of existence by atmospheric pressure. In the video below, Dr. Pasquale uses a hotplate to boil water. You can see that the water turns into a gas within the liquid. Those gas bubbles move upward due to a buoyant force and then leave the beaker.
Boiling has to do with atmospheric pressure. At standard atmospheric pressure and temperatures, a beaker of water is not going to boil. This is because any gas bubbles that try to form will collapse back into the liquid state due to the pressure of the atmosphere pushing down from above. As the liquid heats up, the energy of the bubbles increases, until a certain point where the bubbles overcome atmospheric pressure. This is known as the boiling point.
At a higher elevations, atmospheric pressure is lower. This means that the temperature at which water will freely exist in the gas phase is lower. In fact, if you have ever cooked cake or brownies from a mix, you may have seen a high altitude note on the box. This is because water boils at a lower temperature at high altitudes, and things may not cook as thoroughly as they would at sea level.
It is possible to boil water at room temperature by reducing atmospheric pressure. Dr. Pasquale demonstrated this in the video below using a vacuum chamber. By pumping out most of the surrounding air, there is no longer enough pressure to collapse any bubbles that form in the water. This beaker of water is boiling at room temperature.
This demonstration emphasizes why humans need to wear pressure suits at extremely high altitudes in flight, or in the vacuum of space. Above 19,000 meters, the fluid in our throat and lungs will boil away. A pressure suit compensates for this by compressing the human body, preventing this boiling process.
Latent heat of vaporization
Latent heat of vaporization describes the amount of heat required to convert one gram of a substance from a liquid form to a gaseous form. Alternatively, it defines the amount of heat that must be removed from one gram of a substance to convert it from a gas to a liquid.
During the vaporization or condensation process, it is important to note that the temperature of the substance remains constant. Any heat entering the liquid does not increase the temperature: it changes the molecular arrangement of the atoms. Any heat leaving the substance does not decrease the temperature: it changes the arrangement of the atoms.
Because the vaporization and condensation temperatures are the same, and the amount of latent heat is the same for both processes, we can only tell if a material is vaporizing or condensing by determining if the object is gaining heat or losing heat. If the substance is gaining heat, then the molecules are being reconfigured into a gas and vaporization is occurring. If the substance is losing heat, then the molecules are being reconfigured into a liquid and condensation is occurring.
Water has a relatively high latent heat of vaporization, especially compared to other common substances. The latent heat of vaporization of water is 540 cal/g. This means that 540 calories of heat must be added to one gram of water at 100 oC to convert it into one gram of steam at 100 °C.
The equation we can use for calculating the amount of heat required to change the phase of a substance from liquid to gas is
Heat equals the mass of the substance times its latent heat of vaporization.
Melting and freezing
Melting is the phase change that occurs when a substance converts from a solid to a liquid. Because a liquid has more energy than a solid, that means that energy must be provided to a substance to get it to change its phase.
In the melting process, molecules in their solid state absorb energy. That energy loosens the tight bonds between the molecules, allowing them to move about more freely. Heat is required to convert a solid to a liquid. That heat must come from somewhere. When melting occurs, the surrounding environment cools down after it provides that heat. (Similar to what was discussed above, the surrounding environment is typically large enough not to have its temperature noticeably affected by this process.)
Freezing is the opposite of melting. It is the phase change from a liquid to a solid. As the molecules lose energy in the freezing process, they start to form tight bonds, which gives solids a very well-defined shape. The energy lost by the substance is released into the surroundings, causing them to warm up. Again, the temperature change of the surroundings, which are extremely large with respect to the freezing substance, can typically be ignored. (An example of this would be adding a ladle of liquified molten iron into Lake Michigan. The heat released into Lake Michigan from freezing the molten iron back to its solid form, and then cooling it to the same temperature of the lake, would not be sufficiently high to cause a noticeable change in temperature of the lake.)
It is important to note that, for a given substance, melting and freezing occur at the same temperature. Water will freeze at 0 oC, and it will also melt at 0 oC. Whether it converts from a solid to a liquid, or from a liquid to a solid, depends on whether it is gaining energy from the environment or losing energy to the environment at this temperature.
In the winter, salt is frequently placed on roadways and sidewalks to cause ice to melt. Salt will block the bonds between water molecules, making it much harder for liquid water to freeze into a solid. This lowers the freezing point; salt water has a lower freezing point than fresh water. If the freezing point is lower, the melting point is also lower. Frozen salt water will melt at a lower temperature than fresh water.
Latent heat of fusion
Latent heat of fusion describes the amount of heat required to convert one gram of a substance from a solid form to a liquid form. Alternatively, it defines the amount of heat that must be removed from one gram of a substance to convert it from a liquid to a solid.
During the melting or freezing process, it is important to note that the temperature of the substance remains constant. Any heat entering the solid does not increase the temperature: it changes the molecular arrangement of the atoms. Any heat leaving the liquid does not decrease the temperature: it changes the molecular arrangement of the atoms.
Because the melting and freezing temperatures are the same, and the amount of latent heat is the same for both processes, we can only tell if a material is melting or freezing by determining if the object is gaining heat or losing heat. If the substance is gaining heat, then the molecules are being reconfigured into a liquid and melting is occurring. If the substance is losing heat, then the molecules are being reconfigured into a solid and freezing is occurring.
The latent heat of fusion of water is 80 cal/g. This means that 80 calories of heat must be added to one gram of ice at 0 oC to convert it into one gram of water at 0 °C.
The equation we can use for calculating the amount of heat required to change the phase of a substance from solid to liquid, or from liquid to solid, is
This is similar to an equation discussed earlier in this chapter, but now instead of using the latent heat of vaporization, we use the latent heat of fusion.
Triple point
The triple point is a particular combination of temperature and pressure at which a substance will exist in liquid, solid, and gaseous forms simultaneously. This might seem outrageous, but it is simply an incredibly interesting property of matter. When held at the triple point, both the freezing/melting and condensation/vaporization processes can be seen happening at the same time, with all three phases (solid, liquid, and gas) existing in equilibrium with each other.
The boiling point (and freezing point) of a given substance generally varies with pressure. Recall that the temperature at which water boils decreases when atmospheric pressure is greatly reduced. The triple point is a unique point that exists at a particular temperature and pressure for a substance.
The video below shows a solvent (cyclohexane) at the triple point. As you watch the video, notice how the processes of freezing and boiling occur simultaneously. The solvent exists in three states of matter (solid, liquid, and gas) at the same time.
Sublimation and deposition
Sublimation is the process by which a substance goes directly from a solid to a gas without going through the liquid phase. Some substances, such as carbon dioxide, cannot exist as a liquid at standard atmospheric pressure.
The video below shows a piece of dry ice as it was recorded over a span of half an hour. While you cannot actually see the carbon dioxide when it turns into a gas, it is clear that the piece of dry ice becomes physically smaller over time, and no liquid is generated. The carbon dioxide goes directly from solid to gaseous form.
Water vapor from the surrounding air creates frost on the surface of the dry ice over time. The temperature at which carbon dioxide will sublimate is just about -80 oC, much colder than the freezing point of water!
Therefore, we are not only witnessing the sublimation of carbon dioxide, but the deposition of water. Deposition is the opposite process to sublimation: it is when a gas turns directly into a solid without passing through the liquid form. This occurs all the time in winter when water vapor in the air turns into frost.
Deposition of substances such as chromium, gold, platinum, copper, and other metals is frequently used in the semiconductor industry to create electric contacts, sensors, and other micro-scale or nano-scale electronics and optical devices.
Ionization and recombination
Ionization is the process by which a gas is converted into a plasma. As heat is added to a gas, the energy of the gas increases to such an extent that electrons orbiting the atom are ripped away, causing the gas molecules to have an imbalance of electric charge. Recall from earlier in this textbook that an atom with different numbers of electrons and protons is known as an ion.
Recombination is the reverse process. It occurs when a plasma loses energy and gains its electrons back, converting back to a regular gas.
Phase change diagrams
A phase change diagram shows the relationship between temperature and heat in a substance. The x-axis of the graph shows how much heat is added to a substance. In this textbook, that heat will be measured in calories. Along the y-axis is the temperature of the substance measured in degrees Celsius. From these graphs, we can learn a lot about a substance. We can determine the melting/freezing point, boiling/condensation point, latent heat of fusion, latent heat of vaporization, and specific heat capacity of the solid, liquid, and gaseous forms of the substance.
Figure 17.2 shows an example of a phase change diagram for one gram of a random substance. This particular phase change diagram starts in the lower left corner with a temperature of -200 oC and no heat added. As heat is added, the temperature increases. This increase continues until 50 cal of heat have been added, at which point the temperature hits a plateau at -100 oC. Then the temperature remains constant at -100 oC until an additional 150 cal of heat are added. After that, the temperature starts to increase with more added heat. Adding an additional 150 cal of heat causes the temperature to rise to +100 oC, at which point another plateau is reached. The temperature remains constant through the addition of another 200 cal of heat. Finally, adding 150 cal of heat causes the temperature of the substance to rise from 100 oC to 500 oC.
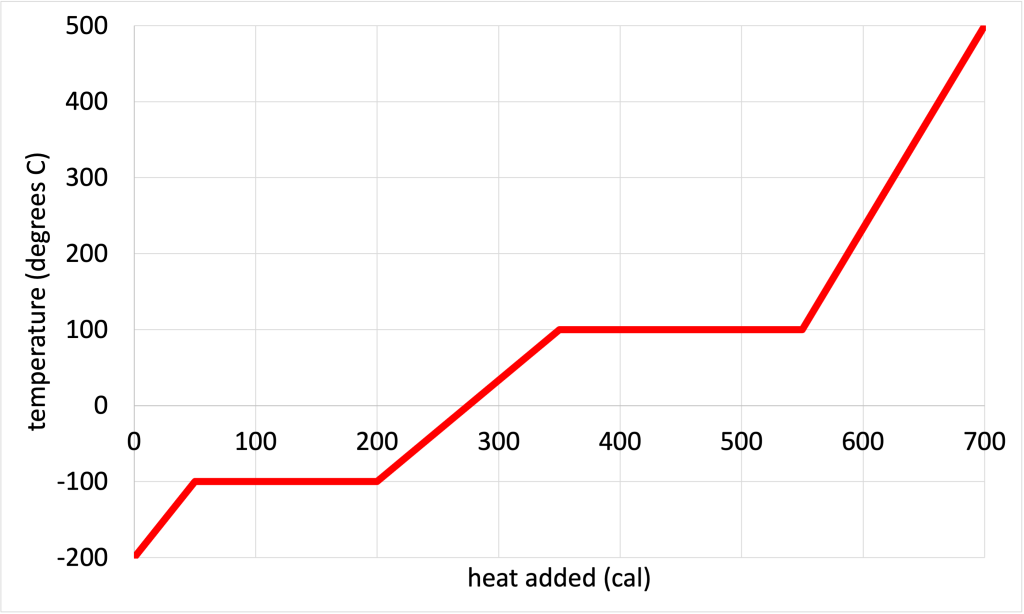
This phase change diagram contains information about the phases and phase changes of the substance. This is shown in Figure 17.3, which has the same phase change diagram as in Figure 17.2, with all of the phases and phase changes labeled. The colder temperatures of the graph represent the substance in its solid form. The middle region represents the substance in liquid form. The hotter region represents the substance in its gaseous form. The melting/freezing point is the colder plateau temperature, and the boiling/condensation point is the hotter plateau temperature. These are phase change temperatures because adding heat doesn’t increase the temperature but rearranges the bonds between the atoms in the substance to change phase.
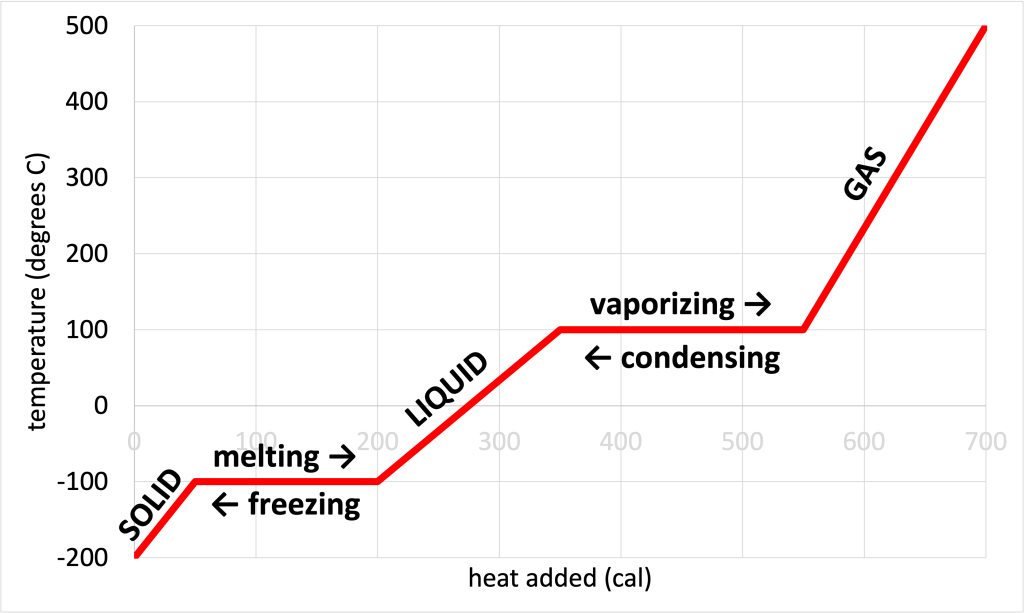
The plateaus also provide information about the latent heats of fusion and vaporization. From this graph, we see that it requires 100 cal of heat to convert the substance from a solid to a liquid, while remaining at a temperature of -100 oC. Therefore, the latent heat of fusion is 100 cal/g. It requires 200 cal of heat to convert the substance from a liquid to a gas at a constant temperature of 100 oC. Therefore, the latent heat of vaporization is 200 cal/g.
The rising areas of the graph give information about how much heat is required to change the temperature of the substance while it is in a single phase – this is related to the specific heat capacity. The equation for specific heat capacity is
Therefore, to calculate the specific heat capacity of the substance in any form, divide the heat added to the substance by the mass of the substance times the temperature change of the substance.
For the phase change diagram shown in Figure 17.2, in the solid phase, 50 cal of heat increases the temperature from -200 oC to -100 oC, a temperature change of 100 Co. The specific heat capacity of the solid form of this substance is 0.5 cal/gCo.
In the liquid phase, 150 cal of heat changes the temperature of the substance from -100 oC to 100 oC, a temperature change of 200 Co. The specific heat capacity of the liquid form of this substance is 0.75 cal/gCo.
In the gaseous phase, 150 cal of heat changes the temperature from 100 oC to 500 oC, a temperature change of 400 Co. The specific heat capacity of the gaseous form of this substance is 0.375cal/gCo.
The phase change diagram can also be used to answer other questions about temperature change and heat added. For example: “how much heat is required to convert 1 gram of this substance from a solid at -100 oC to a gas at 100 oC?” First, identify the two points on the graph. The point where the substance is completely solid at -100 °C is at the start of the plateau representing the latent heat of fusion, before the phase changes to liquid. The point where the substance is completely gaseous at 100 °C is at the end of the plateau representing the latent heat of vaporization, after the phase completely changes to gas. Reading from the x-axis, it requires 450 calories of heat to go from one to the other point.
This process can be repeated to answer other questions. Identify the two points on the graph. Then, use the change in y-axis value to determine the temperature change or the change in x-axis value to determine the required heat added.
Further reading
- The engineering of the drinking bird – This video gives a great explanation of the drinking bird toy. The topics include evaporation, thermodynamics, and rotational motion.
Practice questions
Numerical analysis
- If 100 g of ice at -10 oC is heated until it turns into water at 0 oC, calculate the amount of heat energy that will be absorbed by the ice during this process. (The specific heat capacity of ice is 0.5 cal/goC and the latent heat of fusion of water is 80 cal/g.)
- A pot of water on the stove is boiling vigorously at 100 oC. If you continue to apply heat, calculate how much energy is required to convert 500 g of boiling water into steam at 100 oC. (The latent heat of vaporization of water is 540 cal/g.)
- A scientist wants to melt a 50 g block of lead at its melting point. Calculate the amount of heat energy required to turn the solid lead at 327 oC into liquid lead at 327 oC. (The latent heat of fusion of lead is 5.5 cal/g.)
- For the phase change diagram shown in Figure 17.4 (which depicts the temperature change vs. added heat for one gram of a mystery substance), calculate the…
- …freezing/melting point of the substance.
- …condensation/vaporization point of the substance.
- …latent heat of fusion of the substance.
- …latent heat of vaporization of the substance.
- …specific heat capacity of the solid form of the substance.
- …specific heat capacity of the liquid form of the substance.
- …specific heat capacity of the gaseous form of the substance.
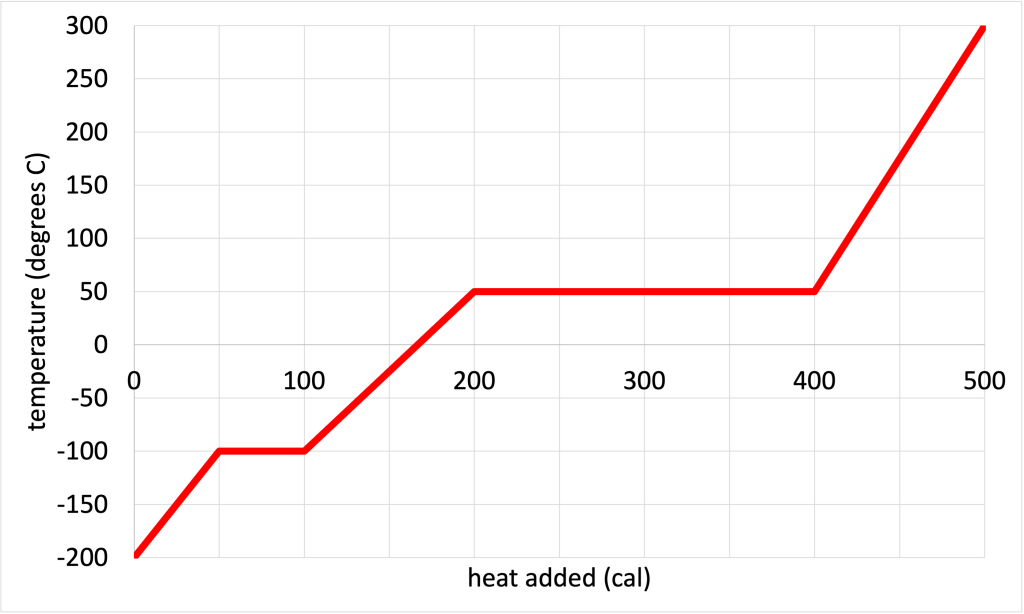
A solid is a substance where the molecules or atoms are very tightly bound together. This gives a solid a very rigid volume and shape. Solid is one of the four most common phases of matter.
A liquid is a state of matter in which the constituent molecules will change their shape or arrangement but cannot be easily compressed to change their volume. Liquid is one of the four most common phases of matter.
Gas is a state of matter where molecules are very free to move about and generally do not interact with each other except during collisions. This means that the shape and volume of a gas is free to change. Gas is one of the four most common phases of matter.
Plasma is ionized gas. Plasma is the most common phase of matter in the universe.
Energy is defined as the capability of an object (or collection of objects) to do useful work. (symbol: E, unit: J)
An electron is a fundamental building block of matter that has a negative charge and is found surrounding the nucleus of an atom.
Temperature defines the average kinetic energy of an object. It quantifies the “hotness” or “coldness” of something. (symbol: T, unit: °C or K)
Absolute zero is the thermodynamic limit corresponding to the lowest possible temperature. Absolute zero is defined to be 0 K.
Pressure defines the amount of force applied over an area of a substance. (symbol: P, unit: Pa)
Quantum physics describes the physical laws used to describe the properties of matter and energy that occur on a very small scale.
A neutron is a subatomic particle that has no charge and resides in the nucleus of an atom.
Vaporization is the phase change that occurs when a substance converts from a liquid to a gas.
Heat is energy that is transferred from one object to another in response to a difference in temperature. (symbol: Q, unit: cal)
Torque is a twist or a turn that causes an object to change its rotational motion. Torque creates rotational acceleration and causes the rotation of an object to speed up, slow down, or change direction. (symbol: τ, unit: Nm)
Condensation is the phase change from a gas to a liquid.
Kinetic energy is the energy that an object has due to its motion. (symbol: KE, unit: J)
Latent heat of vaporization describes the amount of heat required to convert one gram of a substance from a liquid form to a gaseous form. Alternatively, it defines the amount of heat that must be removed from one gram of a substance to convert it from a gas to a liquid.
Mass is a property of physical objects that relates to resistance to changes in motion: inertia. (symbol: m, unit: kg)
Melting is the phase change that occurs when a substance converts from a solid to a liquid.
Freezing is the phase change from a liquid to a solid.
Latent heat of fusion describes the amount of heat required to convert one gram of a substance from a solid form to a liquid form. Alternatively, it defines the amount of heat that must be removed from one gram of a substance to convert it from a liquid to a solid.
Sublimation is the process by which a substance goes directly from a solid to a gas without going through the liquid phase.
Deposition is the phase change process when a gas turns directly into a solid without passing through the liquid form.
Ionization is the process by which a gas is converted into a plasma.
The orbit of an electron describes the properties of the electron wave as it exists around the nucleus of an atom.
Electric charge is a fundamental property of matter that causes particles that carry a charge to experience a force when in the presence of an electric field.
A proton is a subatomic particle that has a positive charge and resides in the nucleus of an atom.
An ion is a charged atom; there is an unequal number of protons and electrons in the atom.
Recombination is the process by which a plasma changes phase to a gas.
Specific heat capacity defines how difficult it is to change the temperature of a substance. It describes the amount of heat required to change the temperature of a certain mass of a substance by a certain temperature. (symbol: c, unit: cal/(g°C))
Thermodynamics is the study of heat flow and its relationship to temperature, work, energy, and entropy.